Advisory note
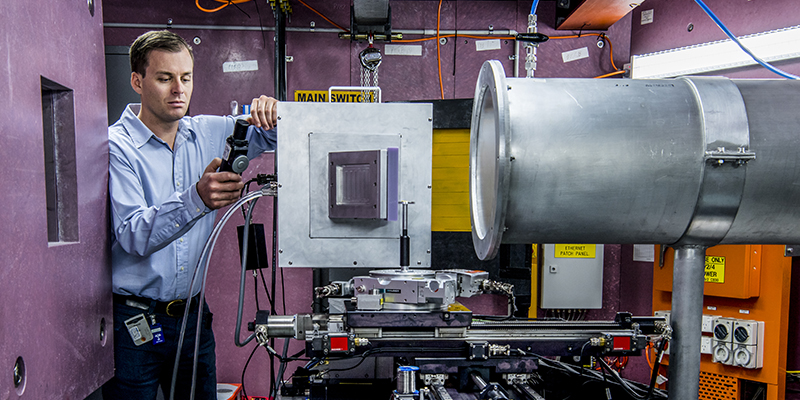
Summary
The International Commission for Radiation Protection (ICRP) is an independent, international organisation that develops and maintains the International System of Radiological Protection. The work produced by the ICRP is published in their reports and is commonly used as a basis for radiation protection standards, legislation, guidelines, programmes and practice.
The ICRP are in the process of publishing a series of new reports on the Occupational Intake of Radionuclides (OIR) that update dose coefficients for occupationally exposed workers. To date, four parts of the five-part series have been published. This series will impact the dose assessment methodology for determining occupational exposures to workers from the intake (inhalation and ingestion) of radioactive materials.
Notes to reader
ARPANSA has previously provided an advisory and additional information in regards to changes for the dose coefficient for radon and radon progeny as published by the ICRP in OIR Part 3.
This advisory is aimed at regulators with an understanding of the radiation protection framework and the use and implementation of dose coefficients. It is aimed to provide information regarding changes to the dose coefficients for all other radionuclides.
Changes in the international publications
The ICRP have published the fourth part of their updated OIR series, the first of which was released in 2015. These documents provide updated dose coefficients for all radionuclides and will replace ICRP Publications 54, 68 and 78 (ICRP, 1988a & 1997b). The series will comprise of five parts:
- Part 1 – Provides a description of biokinetic and dosimetric methodology, and the use of bioassay data (ICRP Publication 130, 2015)
- Part 2 – hydrogen (H), carbon (C), phosphorus (P), sulphur (S), calcium (Ca), iron (Fe), cobalt (Co), zinc (Zn), strontium (Sr), yttrium (Y), zirconium (Zr), niobium (Nb), molybdenum (Mo), and technetium (Tc) (ICRP Publication 134, 2016)
- Part 3 – ruthenium (Ru), antimony (Sb), tellurium (Te), iodine (I), caesium (Cs), barium (Ba), iridium (Ir), lead (Pb), bismuth (Bi), polonium (Po), radon (Rn), radium (Ra), thorium (Th), and uranium (U) (ICRP Publication 137, 2018);
- Part 4 – lanthanum (La), cerium (Ce), praseodymium (Pr), neodymium (Nd), promethium (Pm), samarium (Sm), europium (Eu), gadolinium (Gd), terbium (Tb), dysprosium (Dy), holmium (Ho), erbium (Er), thulium (Tm), ytterbium (Yb), lutetium (Lu), actinium (Ac), protactinium (Pa), neptunium (Np), plutonium (Pu), americium (Am), curium (Cm), berkelium (Bk), californium (Cf), einsteinium (Es), and fermium (Fm) (ICRP Publication 141, 2019); and
- Part 5 – beryllium (Be), fluorine (F), sodium (Na), magnesium (Mg), aluminium (Al), silicon (Si), chlorine (Cl), potassium (K), scandium (Sc), titanium (Ti), vanadium (V), chromium (Cr), manganese (Mn), nickel (Ni), copper (Cu), gallium (Ga), germanium (Ge), arsenic (As), selenium (Se), bromine (Br), rubidium (Rb), rhodium (Rh), palladium (Pd), silver (Ag), cadmium (Cd), indium (In), tin (Sn), hafnium (Hf), tantalum (Ta), tungsten (W), rhenium (Re), osmium (Os), platinum (Pt), gold (Au), mercury (Hg), thallium (Tl), astatine (At), and francium (Fr). Additional dosimetric data for exposure from submersion in a cloud of gas are given in Annex A for the noble gases neon (Ne), argon (Ar), krypton (Kr), and xenon (Xe). (ICRP Publication 151, 2022)
The ICRP’s Committee 2 is responsible for the development of dose coefficients for the assessment of internal and external radiation exposure; development of reference biokinetic and dosimetric models; and reference data for workers and members of the public. The task group working to update dose coefficients has also been assigned to update the anatomical models to those based on information obtained through medical imaging. This aligns with ensuring that the models used for dose assessment are based on the best available body of knowledge as part of the ongoing review process. The task group also updated the nuclear decay data and reviewed and updated, where necessary, all biokinetic models used for the determination of dose coefficients.
Part 1 contains the key information regarding the control of occupational exposures, biokinetic and dosimetric models, monitoring methods, monitoring programmes and retrospective dose assessment. This report provides a description of the changes made to the ICRP reference Human Respiratory Tract Model (HRTM) (ICRP, 1994a) and an overview of the ICRP reference Human Alimentary Tract Model (HATM) (ICRP, 2006).
Parts 2, 3, 4 & 5 contain information for each of the radionuclides including the typical chemical forms found in workplaces, routes of entry, monitoring techniques, dosimetric data and key references. The documents published to date do not provide complete dosimetric data for all radionuclides or dose integration times and are limited to gases/vapour and particles, specifically particles with a 5 m activity median aerodynamic diameter (AMAD) and 50 year integration time. Comprehensive information has been made available in electronic annexes which contain the information for all isotopes and varying particle sizes. The electronic annexes for OIR Part 2, 3, 4 & 5 are available from the ICRP publications website.
Advice to implement changes
Regulators are advised to review the above documents and associated annexes against their licence holders monitoring programs and dose assessment methodologies. They should decide on an implementation plan for changes from currently used dose coefficients to ones published in this series. Changes should be considered as soon as the new data for the relevant radionuclides is available.
Relevance to industries handling or processing Naturally Occurring Radioactive Materials (NORM)
Comprehensive data for the uranium and thorium decay series is now available. Regulators with active uranium and other naturally occurring radioactive material (NORM) mines should review the dose coefficients.
It has previously been recognised that the information provided for dose coefficients in Table 1 of the Code of Practice for Radiation Protection and Radioactive Waste Management in Mining and Mineral Processing (RPS9) has not been consistently derived and the results have been calculated for different radon retention percentages. This has resulted in calculations demonstrated in the Safety Guide Monitoring, Assessment and Recording Occupational Radiation Doses in Mining and Mineral Processing (RPS 9.1) also being inconsistent. ARPANSA has previously provided advice to address the inconsistency with the dose coefficient values in RPS 9. Reference is made to the Western Australian document Managing Naturally Occurring Radioactive Material (NORM) in Mining and Mineral Processing – Guideline NORM 5, Dose Assessment, Department of Mines and petroleum, (2010) The publication of OIR Part 4, completing the dose coefficients for the uranium and thorium decay series, now makes the dose coefficients provided in RPS 9, RPS 9.1 and the WA Guideline NORM 5 redundant.
Work has commenced to update the ARPANSA owned documents RPS 9 and RPS 9.1. In the interim ARPANSA has revised Tables 1 and 2 from RPS 9 and Tables A1 and A2 from RPS 9.1. This revised information provides new dose coefficients for radon, mixtures of radionuclides, uranium and thorium decay series. This updated information is included as an amendment to RPS 9 & 9.1.
Medical and other sectors
Dose assessment programs in the medical and other sectors may require the consideration of doses arising from the intake of radionuclides primarily in radiopharmaceutical production and administration of gaseous or volatile radionuclides. As a result, the use of occupational dose coefficients in these sectors is generally limited to doses occurring as a result of incidents. Regulators and radiation safety officers should be aware of the publication of these new dose coefficients and use appropriate factors when determining relevant occupational exposures. Not all radionuclides used in the medical or other sectors have been covered in the publications to date and further assessment in these sectors will await publication of OIR Part 5.
Estimation of Doses: Uncertainties and Errors
Dose conversion factors are but one component of the calculations that are used to provide a dose estimate. The estimations for the inhalation, ingestion or injection doses of radionuclides requires using a range of assumptions and sampling techniques that all contain a variety of uncertainties and errors. Where actual values are unknown the assumptions used, as taken from scientific literature, always take a conservative approach to ensure that doses are not underestimated.
For example, the concentration of long-lived radioactive dust (LLRD) in the air is derived from collecting representative samples from a statistically significant portion of occupationally exposed personnel performing similar tasks with similar exposures, similar exposure groups (SEG). Sampling is performed using a device that complies with International Standards Organisation (ISO) sampling for inhalable dusts and methodology that complies with the Australian Standard: Workplace Atmospheres – Method for sampling and gravimetric determination of inhalable dust (AS 3640 – 2009). These devices and methods have a range of uncertainties and errors that are mainly associated with the flow rates.
Weighing and gross alpha counting of collected filters allows for the determination of the activity concentration of LLRD in the air. This method is subject to alpha attenuation in samples with heavy dust loadings and correction factors may be applied. This introduces a range of weighing, counting and attenuation errors into the estimation.
Average atmospheric activity concentrations over periods of time (typically a month, quarter or year) within a SEG are used to assign individual doses to members of the SEG. The application of averages to individuals further leads to uncertainties and errors in the dose estimation.
The breathing rate (BR) originates from the ICRP human respiratory tract model and is based upon a Caucasian adult reference male who is a nose breather performing a mix of light work and at rest (5.5 h light exercise + 2.5 h at rest) equating to 9.6 m3 intake of air in 8 h or a breathing rate of 1.2 m3/h. The breathing rate of an individual is primarily dependent on their body size and physical activity and can range significantly for an individual and between individuals. An assumption of a consistent breathing rate can vary doses by a factor of two compared to the actual dose received.
An alternative example for ingestion or injection doses is that they are typically determined from the activity concentrations of radionuclides sampled from excreta by the exposed individual over a period after the exposure occurred. The calculations used to determine the dose make broad assumptions about an individual’s metabolism, hydration, and excretion rates, where these assumptions vary from the individual’s actual status it can result in significant differences to the actual dose received. Even minor errors associated with determination of the actual exposure time can result in large errors for short lived radionuclides.
In general, the DCF is simply one value, based upon best practice assumptions, used in the calculation of doses, a calculation that contains multiple uncertainties, errors, and assumptions. Variations to DCFs to align them with the best available current information that changes them by factors of 2-3 must be kept in context with regards to the other uncertainties and errors that exist in the assumptions and methodologies that are used to estimate doses.